In our attempts to study atmospheric processes, we use several different types of instruments or data, many of which we improved, adapted or developed ourselves. These instruments are stationed in the lab, or in the field on ground-, air-, or space-based platforms. We also use various atmospheric models to help us analyze these observations. Some of these measurement and modeling techniques are discussed below.
The TD-LIF Technique
Reactive nitrogen oxides, including NO, NO2, peroxy nitrates (RO2NO2), alkyl nitrates (RONO2), and nitric acid (HNO3), are key species in the production of ozone (O3), a pollutant dangerous to human health, and in determining the abundance of hydroxyl radical (OH), thus largely controlling the oxidative capacity of the atmosphere. Nitrogen oxides are also influential in regional ecosystem nutrient cycling through nitrogen deposition and are important in the formation of secondary organic aerosol (SOA). Nitrogen oxide emissions to the troposphere occur primarily as NOx (NO + NO2). As these emissions oxidize they form the higher oxides RO2NO2, RONO2, and HNO3. Knowledge of the partitioning of the total nitrogen oxide reservoir between these species thus offers a wealth of information about mechanisms of atmospheric chemistry.
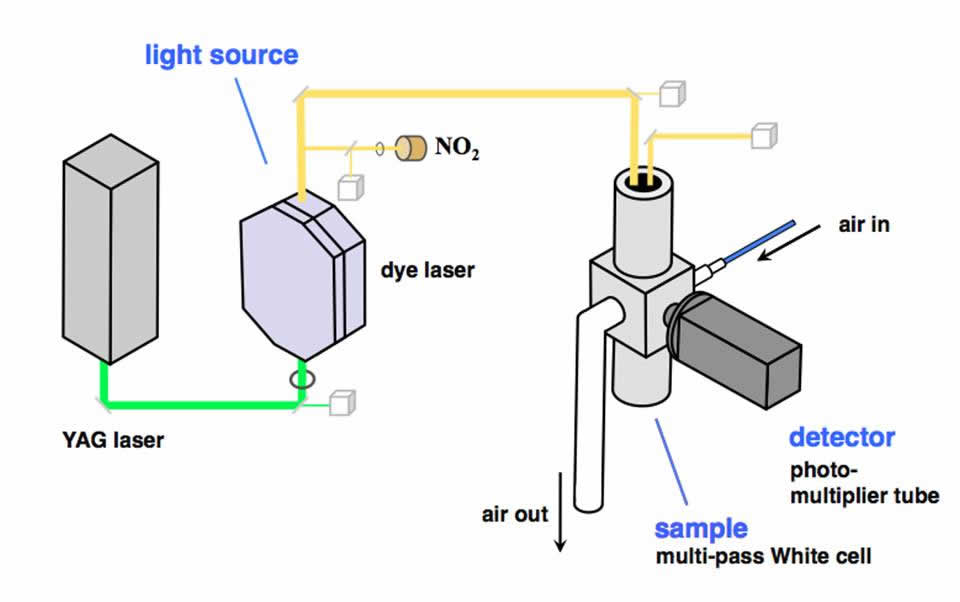
In the Cohen Research Team, we use a technique named thermal dissociation-laser induced fluorescence (TD-LIF) to measure NO2, total RO2NO2 (ΣPNs), total RONO2 (ΣANs), and HNO3. To do this, we couple our ability to detect NO2 at part-per-trillion levels using LIF1 with thermal dissociation (TD). This is possible because the NO2 functionality of RO2NO2, RONO2, and HNO3 is thermally labile at distinct and characteristic temperatures (200°C for ΣPNs, 350°C, for ΣANs, and 600°C for HNO3).2 For over a decade, our TD-LIF instruments have collected nitrogen oxide observations from onboard research aircraft and from ground sites across North America.3 Our ground-based TD-LIF work has included vertical concentration gradients within and above forest canopies and eddy-covariance flux measurements. Please see our publications page to learn more about the wide variety of analyses we have done with our TD-LIF datasets.
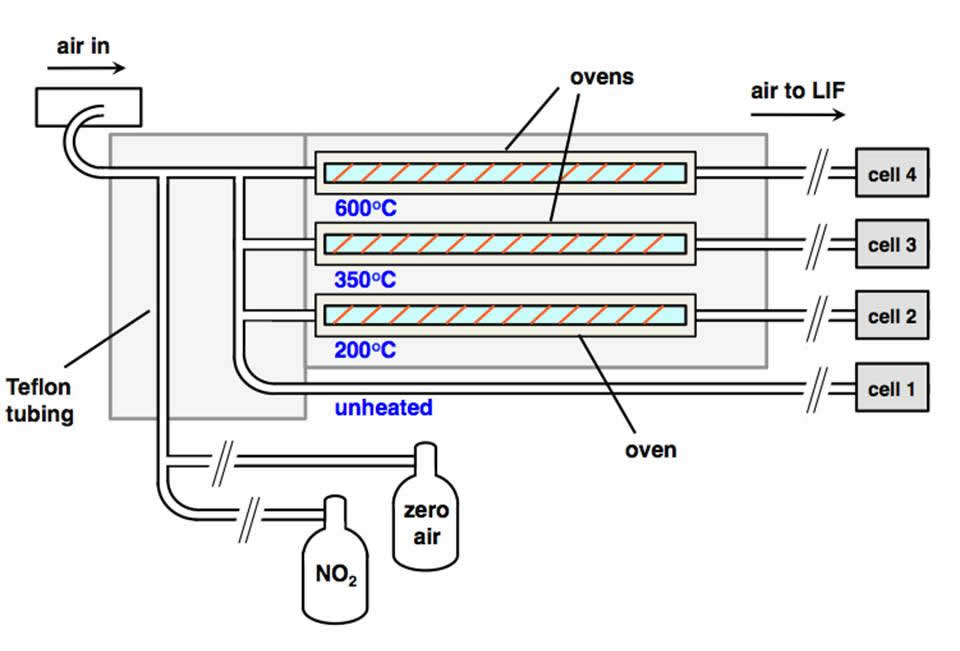
References:
1. J. A. Thornton, P. J. Wooldridge, and R. C. Cohen, Atmospheric NO2: In Situ Laser-Induced Fluorescence Detection at Parts per Trillion Mixing Ratios, Anal. Chem. 72, 528–539, 2000.
2. D. A. Day, P. J. Wooldridge, M. B. Dillon, J. A. Thornton, and R. C. Cohen, A thermal dissociation laser-induced fluorescence instrument for in situ detection of NO2, peroxy nitrates, alkyl nitrates, and HNO3, J. Geophys. Res. 107, 4046, 2002.
3. P. J. Wooldridge, A. E. Perring, T. H. Bertram, F. M. Flocke, J. M. Roberts, H. B. Singh, L. G. Huey, J. A. Thornton, G. M. Wolfe, J. G. Murphy, J. L. Fry, A. W. Rollins, B. W. LaFranchi, and R. C. Cohen, Total Peroxy Nitrates (ΣPNs) in the atmosphere: the Thermal Dissociation-Laser Induced Fluorescence (TD-LIF) technique and comparisons to speciated PAN measurements, Atmos. Meas. Tech. 3, 593-607, 2010.
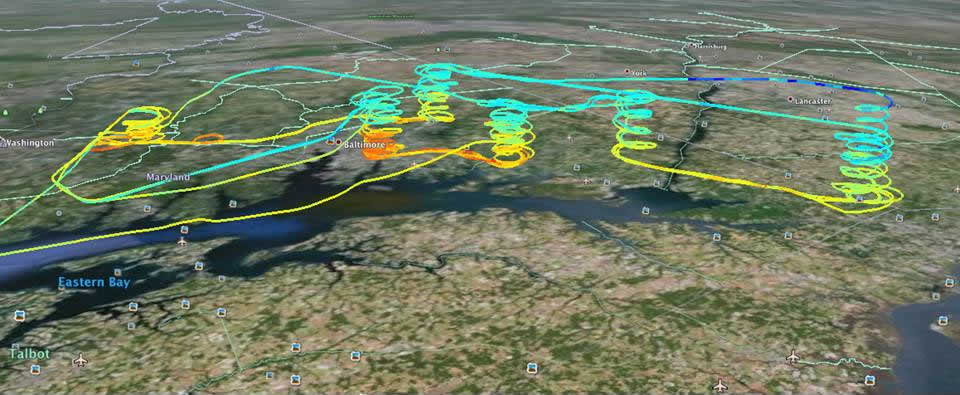
TD-LIF adapted to measure particulate and speciated organic nitrates
We have adapted the already-powerful TD-LIF technique to address questions such as the quantification of speciated organic nitrates as well as the presence of organic nitrates in atmospheric aerosol.
The TD-LIF technique alone provides quantitative information on the total concentration of the chemical class of alkyl nitrates. However, identification and quantification of individual compounds within this class is desirable; to achieve this requires a separation technique. We have coupled gas chromatography with the TD-LIF technique for quantification of individual organic nitrates, detected with unit sensitivity. A second detector (helium ionization detector, VUV ionization) running in parallel provides reference information as well as quantification for compounds that do not contain a nitrate group. In addition to direct sample introduction with a syringe, a specialized inlet system has been designed to directly sample and pre-concentrate gas phase mixtures, aiming for in-situ measurements.
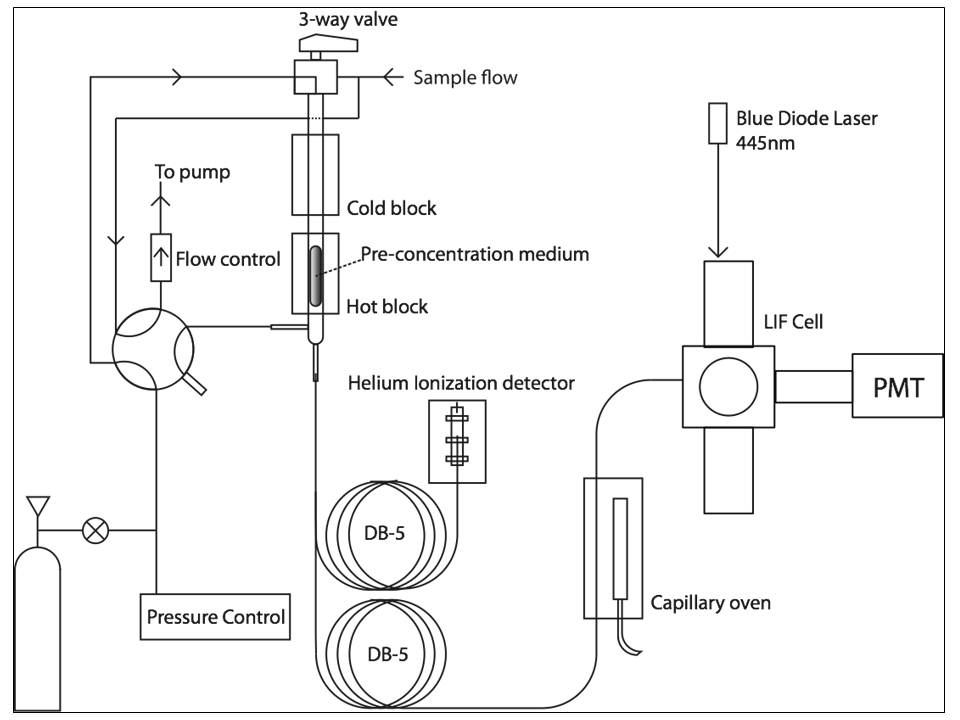
NOx also plays an important and uncertain role in the formation of SOA; as volatile organic compounds are oxidized by NOx, their vapor pressure decreases and they can partition into the particle phase. Another goal, then, is to be able to quantify organic nitrates present in SOA. Coupling an activated charcoal denuder to our TD-LIF system inlet allows us to scrub out gas phase NO2 and organic nitrates and detect only nitrates in aerosol particles, using the difference in diffusion constant between molecules and aerosol particles. This technique has been used to estimate the SOA-forming potential of organic nitrates on the basis of gas phase-condensed phase partitioning when parallel measurements of total organic nitrates (gas+particle) are performed.
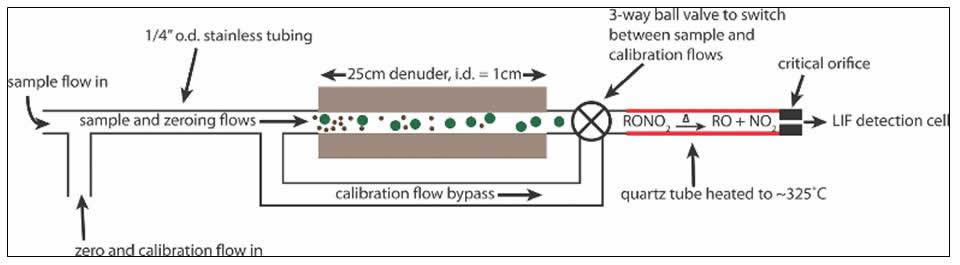
Sensor Network Nodes for Urban GHG and AQ Monitoring
Studies of air quality have often relied on point measurements from a single, highly precise (and thus expensive) instrument. However, high quality measurements can in principle be achieved by a collection of less-expensive instruments with lower individual precision; the mean of this set of observations will have reduced noise (and thus increased precision) and likely will provide a more representative sampling of the area of interest.
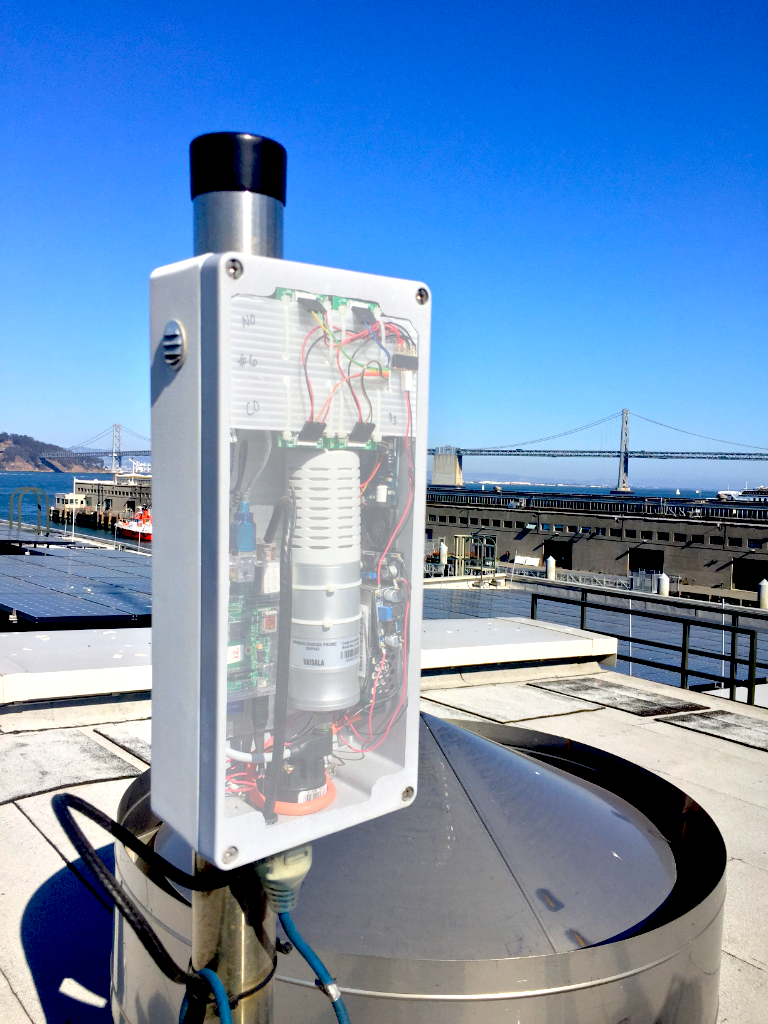
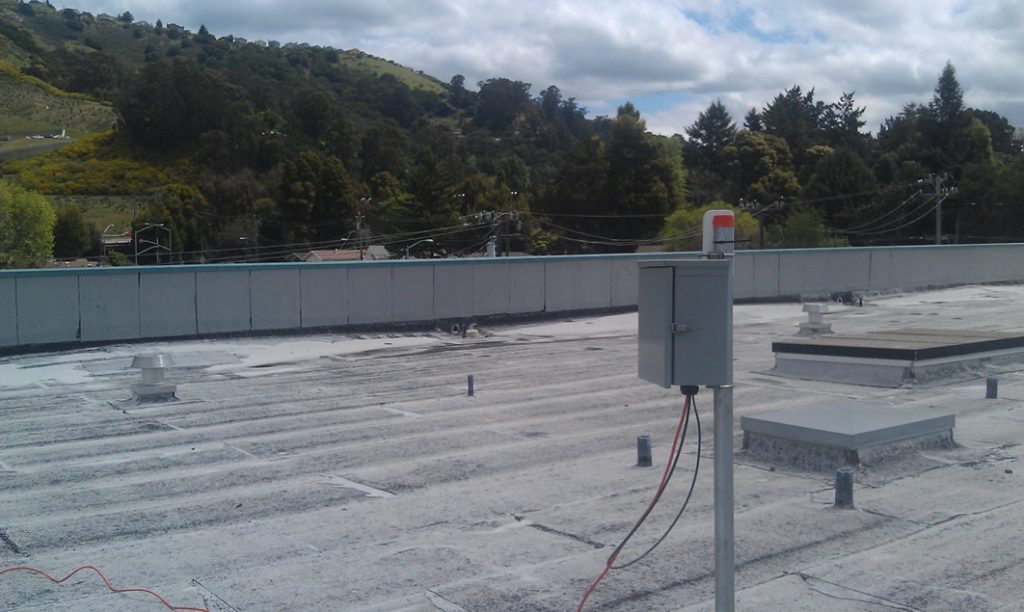
In pursuit of this strategy, we have developed novel sensor “nodes” for use in the BEACON project. Each BEACON site hosts a single node, or sensor package. The node contains inexpensive, commercially available sensors for CO2, NO2, O3, and CO, as well as temperature, pressure, and relative humidity. Data from these sensors are collected once every five seconds onto a miniature computer; data is sent to a server and can also be stored onboard the instrument as necessary. Each BEACON node is contained in a stainless steel box, weighs approximately 25 pounds, and is the size of a backpack. When deployed, the nodes are placed on rooftops, mounted on poles or railing that raise the instruments several feet above the roof surface; care is taken to ensure that the nodes are placed where they will sample free flowing air, away from air handling units or building exhaust. The combination of data from multiple nodes can be used to produce concentration maps, track pollution plumes, or to constrain calculations of emissions, to name a few possibilities.
Satellite Observations
In situ measurements usually have very high spatial and temporal resolution and make very precise measurements; however, these measurements usually suffer from a lack of spatial and temporal coverage, as it is usually logistically and financially impractical to operate multiple instruments over long time scales. Satellite instruments, however, can provide observations on generally much longer time scales with reasonable frequency (~5-15 minutes for instruments on geostationary satellites, up to ~1-3 days for polar-orbiting satellites), at the sacrifice of some of the precision and spatiotemporal resolution of conventional in situ measurements. We use satellite-based observations of NO2 column densities (molecules cm-2) from the Ozone Monitoring Instrument (OMI) to assess long-term regional and global-scale trends and patterns, and provide observational constraints to results from chemical transport models.
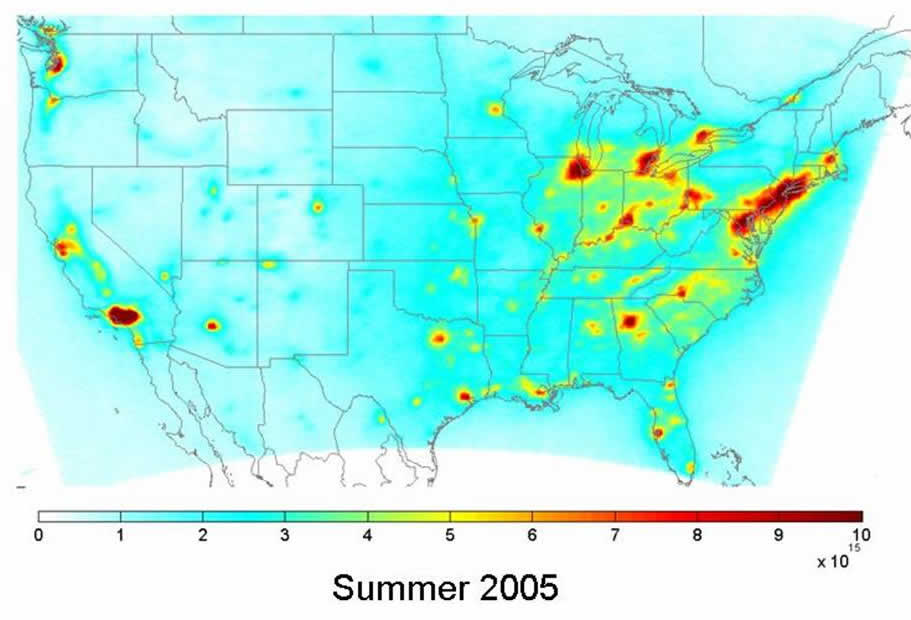
OMI is a UV-Vis spectrometer stationed on the NASA EOS-Aura satellite. OMI uses backscattered radiation from earth in combination with incident sunlight to determine the concentration of ozone-relevant trace gases such as NO2along the light path from the satellite to the ground. To determine the vertical column density over a given location, OMI relies on a priori information about e.g. the surface reflectivity and terrain height, trace gas vertical distribution, and the presence of clouds. We use operational retrievals of OMI NO2 column densities from NASA (the Standard Product or SP) as well as from KNMI (the DOMINO product), which are produced globally and rely on a priori information of moderate resolution. We have also developed our own retrieval of OMI NO2 tropospheric column densities, the BErkeley High Resolution (BEHR) retrieval. This retrieval is applied only to column densities over the United States, and relies on much higher resolution a priori information. To access this data, and for more information about analyses using OMI NO2observations in our group, please check out the BEHR website.
Reference:
A. R. Russell, A. E. Perring, L. C. Valin, E. J. Bucsela, E. C. Browne, P. J. Wooldridge, and R. C. Cohen, A high spatial resolution retrieval of NO2 column densities from OMI: method and evaluation, Atmos. Chem. Phys. 11, 8543-8554, 2011.
Atmospheric Models
The coupling of chemistry and meteorology makes the atmosphere a very complicated place; interpretation of many of our measurements requires some sort of model. These models can be specific to the experiment (e.g. a forest canopy exchange model) or more generalized, and can range from 0-D or 1-D box models with a few chemical species and limited production and loss processes up to global-scale chemical transport models fully coupled to meteorology. We develop simple models on a case-by-case basis, and have developed several experiment-specific models for various purposes (please see our publications page for some examples). We use two models that were developed by other groups for several analyses: GEOS-Chem, a chemical transport model driven by meteorology from the Goddard Earth Observing System (GEOS), for global analyses, and the Weather Research and Forecasting (WRF) model coupled with chemistry (WRF-Chem) for regional-scale analyses. While these models can be used to help interpret data, we have also focused some efforts on highlighting some model weaknesses and making improvements to the models through development of different emissions and chemistry schemes. For example, our work with GEOS-Chem includes development and implementation of a new soil NOx emission scheme that better reflects soil NOx emission dependence on temperature and precipitation when compared with satellite observations (see the figure below left),1 and the demonstration that inclusion of methyl peroxy nitrate chemistry in the model has significant effects on modeled concentrations of NOx and other chemicals.2
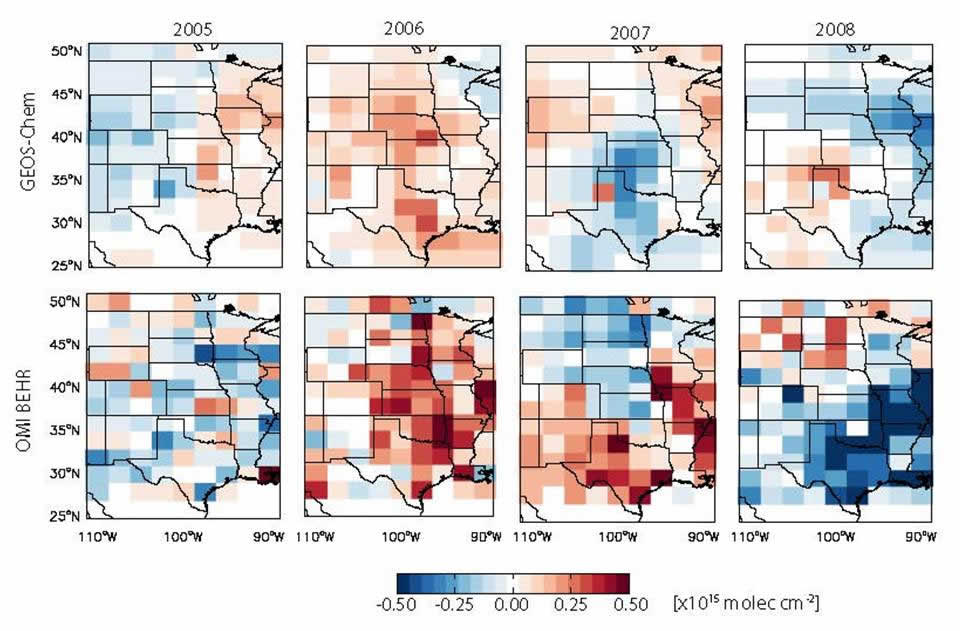
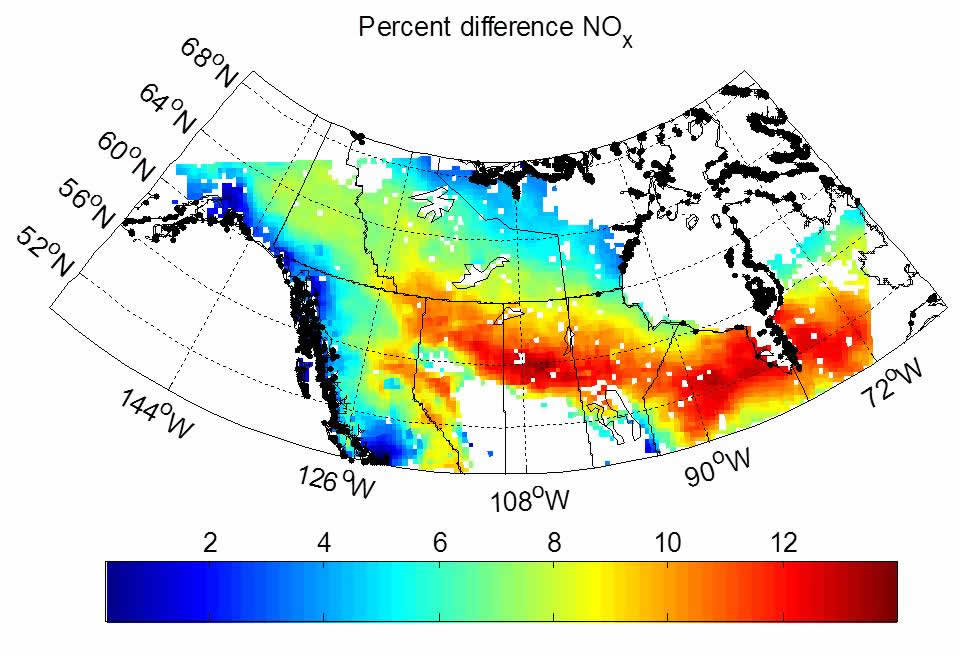
WRF-Chem is selected for regional-scale analyses due to its finer spatial resolution (Δx ≥ 1 km), which improves the treatment of nonlinear chemical processes, and its “online” computation of chemistry, which enables us to consider the feedbacks of chemistry on regional climate. We have developed a more realistic parameterization of organic nitrate chemistry that treats monofunctional, multifunctional saturated, multifunctional unsaturated, aromatic, isoprene, and monoterpene nitrates as unique model species with appropriate lifetimes, and incorporated this chemistry into WRF-Chem (the mechanism can be found here).3 We find that our new extended chemistry predicts the organic nitrate concentration well when compared to observations from ARCTAS and confirms that the organic nitrates are most likely biogenic in origin with a substantial fraction due to monoterpene chemistry. Allowing the monoterpene nitrates to act either as a permanent NOx sink or entirely as a temporary NOx reservoir, we find the difference between these two assumptions is on the order of 10% for both OH and NOx (see the figure above right) over the boreal forest of Canada.
WRF-Chem is also well-suited for the interpretation of space-based measurements of atmospheric composition. The relationship is synergistic – models provide spatially and temporally detailed information on atmospheric composition useful for interpreting measurements that are integrated along the path of reflected sunlight3 while the observations provide critical information on model processes such as emissions and chemistry. For example, spatially-detailed (13 × 24 km2) satellite-based measurements of NO2 provide extraordinary information on the day-of-week patterns of NOx emissions and chemical removal. Synergistically, WRF-Chem provides information on whether any of these processes could possibly bias the NO2 concentration retrieved from the measurements (e.g., does the rate of NOx emissions in a model affect the simulated NO2 vertical profile?).
References:
1. R. C. Hudman, N. E. Moore, A. K. Mebust, R. V. Martin, A. R. Russell, L. C. Valin, and R. C. Cohen, Steps towards a mechanistic model of global soil nitric oxide emissions: implementation and space based-constraints, Atmos. Chem. Phys. 12, 7779-7795, 2012.
2. E. C. Browne, A. E. Perring, P. J. Wooldridge, E. Apel, S. R. Hall, L. G. Huey, J. Mao, K. M. Spencer, J. M. St. Clair, A. J. Weinheimer, A. Wisthaler, and R. C. Cohen, Global and regional effects of the photochemistry of CH3O2NO2: evidence from ARCTAS, Atmos. Chem. Phys. 11, 4209-4219, 2011.
3. E. C. Browne, Observational constraints on the photochemistry of non-acyl peroxy nitrates and organic nitrates on regional and global scales, Ph.D. dissertation, University of California, Berkeley, USA, 2012.
4. A. R. Russell, A. E. Perring, L. C. Valin, E. J. Bucsela, E. C. Browne, P. J. Wooldridge, and R. C. Cohen, A high spatial resolution retrieval of NO2 column densities from OMI: method and evaluation, Atmos. Chem. Phys. 11, 8543-8554, 2011.